Inflammation imaging
Our research efforts in the realm of inflammation imaging have been focused on harnessing the power of fluorine-19 (19F) MRI. Inflammation, recognized as a key driver of numerous diseases, poses a significant challenge in terms of visualization using conventional MRI techniques. In response to this challenge, we have explored various molecular imaging approaches, and our endeavors have led us to the unique capabilities offered by 19F MRI.
Here, we directly image the 19F nucleus instead of the hydrogen (1H) in water. Since there is no naturally detectable 19F in the body, an injected tracer will generate a directly proportional signal. These tracers are tailored nanoparticles filled with perfluorocarbons (PFC), which are safe and inert molecules that are readily taken up by circulating immune cells. This uptake and the proportional signal enable the direct quantification and monitoring of inflammation over time with exceptional specificity even at low cell concentrations (Figure 1).1 These advantages together help us gain a deeper understanding of inflammatory processes within the body.
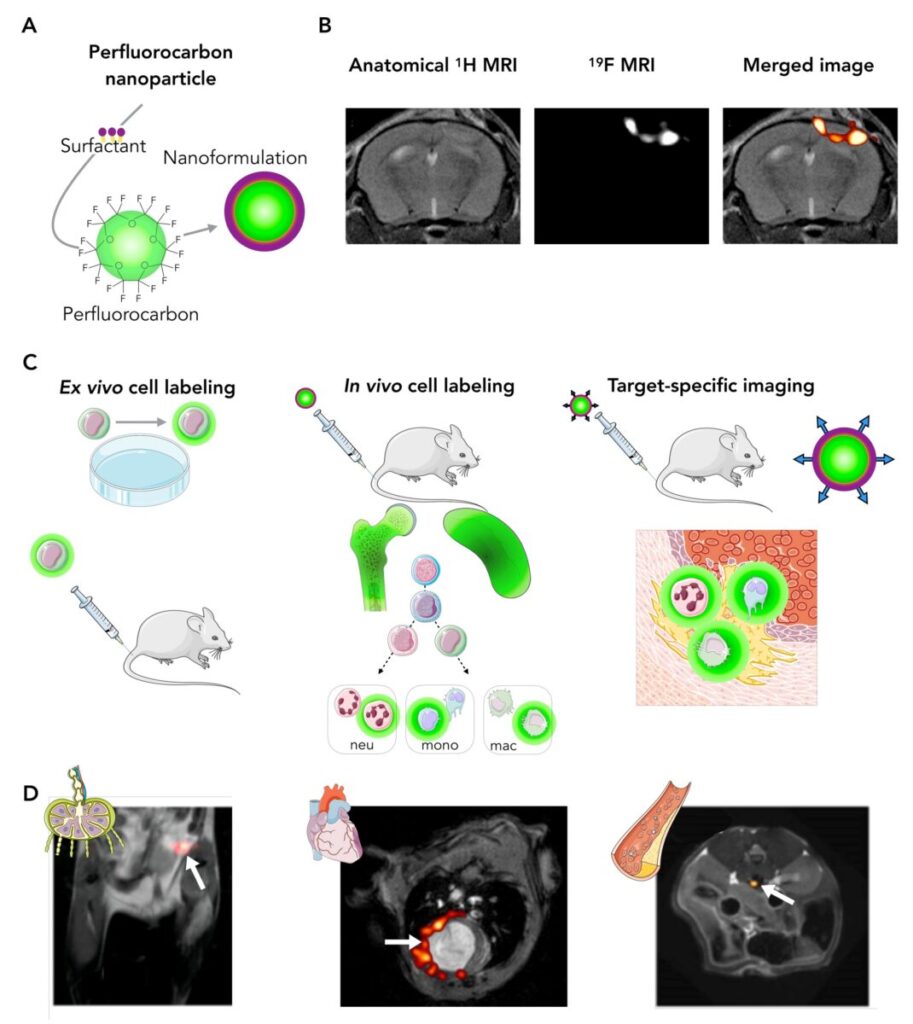
Figure 1. The principles and applications of 19F MRI. A, Hydrophobic perfluorocarbon molecules are packed in tracer nanoparticles. B, 19F MR images can be merged with 1H reference images for anatomic colocalization. C, perfluorocarbon tracers can be used for ex vivo cell labeling, in vivo cell labeling (such as inflammation imaging), or target specific imaging. D, Examples of these strategies include tracking the fate of specific cell populations as they enter lymph nodes (left),quantifying the inflammation burden after a myocardial infarction (middle) or visualizing the exact location of a thrombus (right). Adapted with permission from Van Heeswijk et al.1
Our commitment to advancing the field of inflammation imaging has been recognized through the receipt of several grants from the Swiss National Science Foundation (SNSF grants PZ00P3_154719 and 32003B_182615) and Swiss Heart Foundation (grant FF23129). These have fueled our development of 19F MRI techniques and its characterization in several disease models, mostly in the context of cardiovascular diseases, with the ultimate goal of translating our findings into a first-in-man application to map inflammation.
Imaging of animal models
Our 19F journey began with the exploration of angiography,2 since the lack of background signal should also lead to highly conspicuous vessel depiction (Figure 2). Next, we characterized the sensitivity of 19F in a mouse of myocarditis, where the inflammation load correlated with the histology and the disease degree even before it affected cardiac function.3 In a mouse model of atherosclerosis,4 inflammation in plaques on the order of 1 nL were quantified; first on an animal 9.4T scanner, but later also on a clinical 3T scanner.5
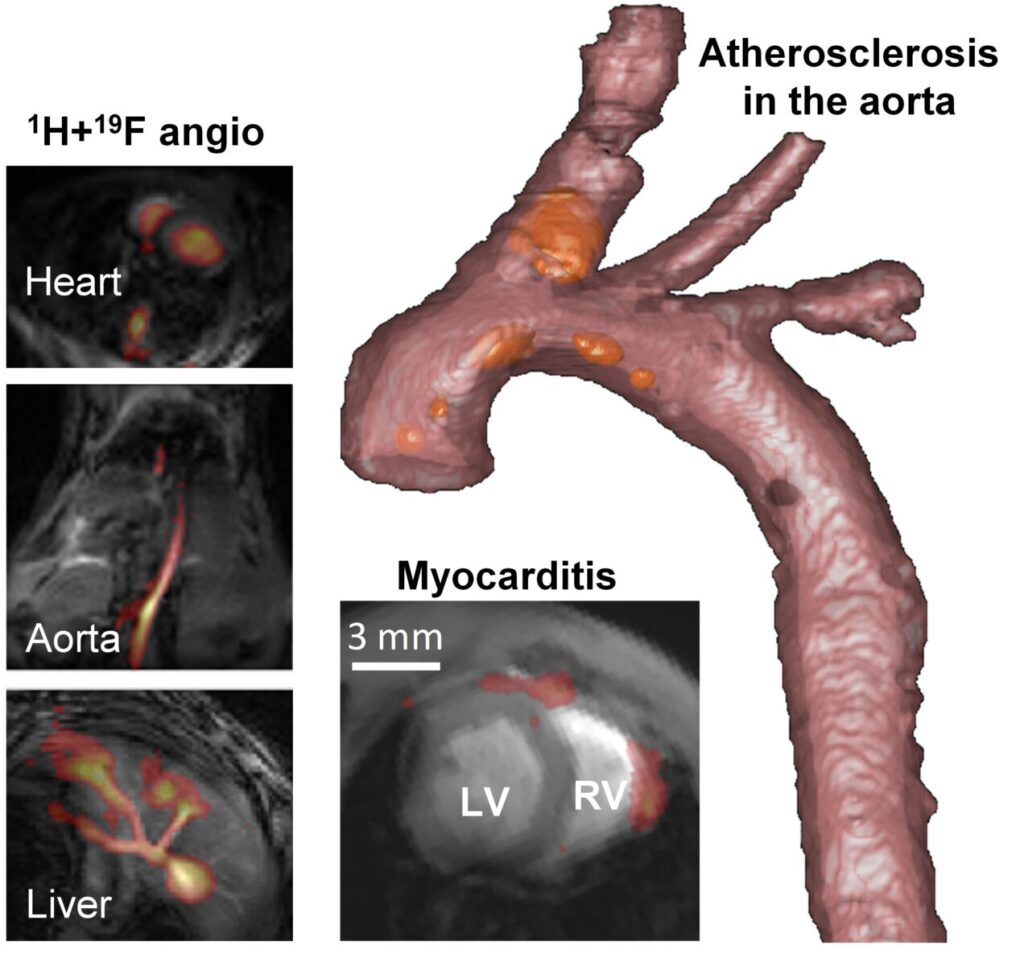
Figure 2. Applications of 19F MRI in mouse models of cardiovascular disease. Left: imaging soon after injection allow for angiography in various organs. Bottom: 19F MRI can be used to visualize inflammation even in the thin right-ventricular wall in a mouse model of auto-immune myocarditis. Right: High-resolution rendering of plaque inflammation in a mouse model of atherosclerosis. The 19F hotspots (in orange) are on the order of a nanoliter.
Collaboration with biologists and physicians is a cornerstone of our research approach, which also resulted in a successful multidisciplinary study together with the Department of Oncology of the CHUV.6 In this study we successfully demonstrated that different immune cell populations can be followed over time before and after radiotherapy in a mouse model of glioma.
This interdisciplinary approach has enriched our understanding of inflammation across various medical domains, providing a holistic perspective on the applications of 19F MRI.
More precise and robust imaging
We are simultaneously interested in increasing the precision and robustness of 19F MRI by optimizing existing techniques7 and by incorporating new technological developments such as chemical shift encoding (CSE)8,9 and compressed sensing (CS, Figure 3).10 This for example enables the imaging of PFCs with complex spectra (but with favorable biological properties) and the visualization of lower concentration or acceleration of the acquisition.
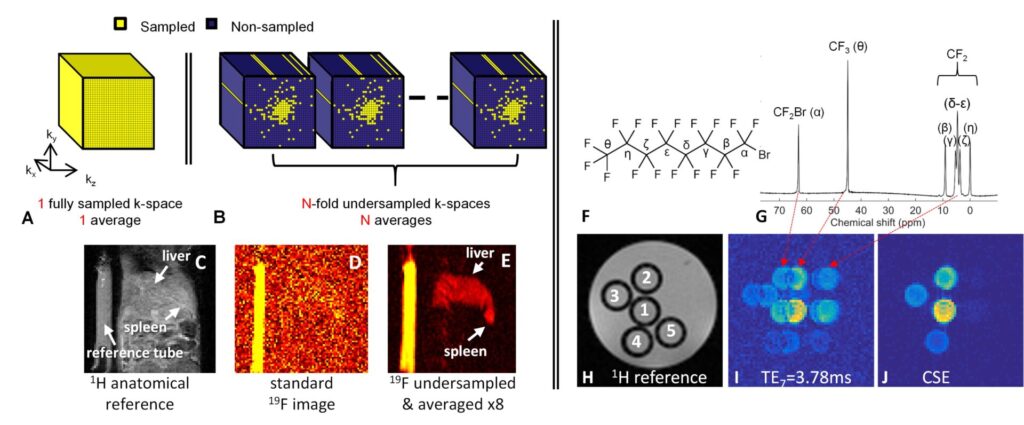
Figure 3. Techniques to improve 19F MR robustness and sensitivity. A-E. Compressed sensing combined with signal averaging allows for a significant increase in sensitivity. F-J. Encoding the known chemical shifts of the various resonances of a PFC like perfluorooctylbromide (F) allows sharp images without chemical shift artifacts to be produced (J).
Translation to the clinic
Given this unique potential for inflammation quantification and monitoring, our recent efforts have focused on translating 19F MRI to the clinical setting together with Aurum Biosciences Ltd.11 Supported by the SNSF, our clinical trial focuses on the non-invasive quantification of inflammation within atherosclerotic plaques in the carotid artery. This objective not only furnishes us with the substrate for future technical, biological, and medical challenges, also lays the foundation for potential breakthroughs in the years to come (Figure 4).
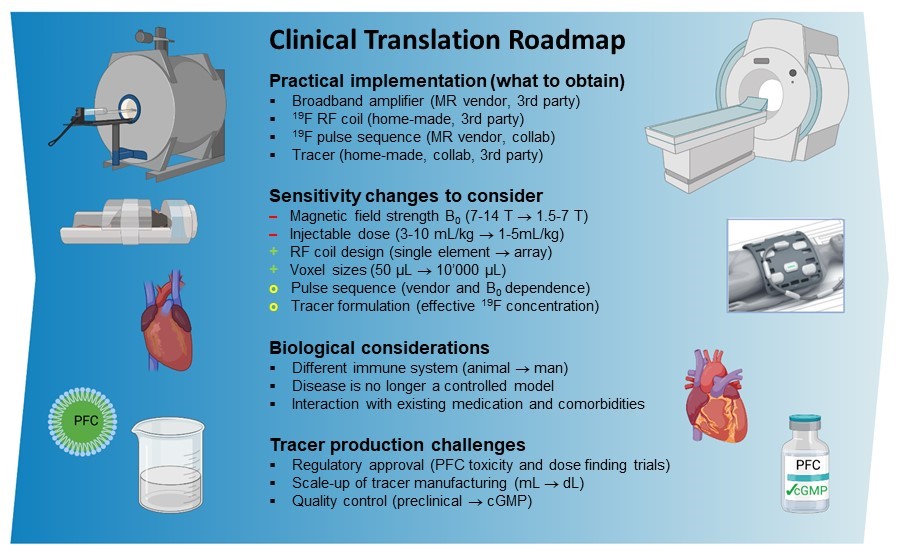
Figure 4. A roadmap for a successful translation of 19F MRI to a clinical diagnostic modality. The practical implementation of 19F MRI into an existing research program is straightforward and can mostly be effected straight away when taking expected sensitivity and biological changes into account. The production of a tracer with regulatory approval is the main remaining challenge. Adapted from van Heeswijk et al.1 with permission.
References
1. Van Heeswijk RB, Bauer WR, Bönner F, Janjic JM, Mulder WJM, Schreiber LM, Schwitter J, Flögel U. Cardiovascular Molecular Imaging With Fluorine-19 MRI: The Road to the Clinic. Circ Cardiovasc Imaging [Internet]. 2023 [cited 2023 Dec 18];16. Available from: https://www.ahajournals.org/doi/10.1161/CIRCIMAGING.123.014742
2. van Heeswijk RB, Pilloud Y, Flogel U, Schwitter J, Stuber M. Fluorine-19 magnetic resonance angiography of the mouse. PloS One. 2012;7:e42236.
3. van Heeswijk RB, De Blois J, Kania G, Gonzales C, Blyszczuk P, Stuber M, Eriksson U, Schwitter J. Selective in vivo visualization of immune-cell infiltration in a mouse model of autoimmune myocarditis by fluorine-19 cardiac magnetic resonance. Circ Cardiovasc Imaging. 2013;6:277–84.
4. van Heeswijk RB, Pellegrin M, Flogel U, Gonzales C, Aubert JF, Mazzolai L, Schwitter J, Stuber M. Fluorine MR Imaging of Inflammation in Atherosclerotic Plaque in Vivo. Radiology. 2015;275:421–9.
5. Darçot E, Colotti R, Pellegrin M, Wilson A, Siegert S, Bouzourene K, Yerly J, Mazzolai L, Stuber M, van Heeswijk RB. Towards Quantification of Inflammation in Atherosclerotic Plaque in the Clinic – Characterization and Optimization of Fluorine-19 MRI in Mice at 3 T. Sci Rep. 2019;9:17488.
6. Croci D, Santalla Méndez R, Temme S, Soukup K, Fournier N, Zomer A, Colotti R, Wischnewski V, Flögel U, van Heeswijk RB*, Joyce JA*. Multispectral fluorine-19 MRI enables longitudinal and noninvasive monitoring of tumor-associated macrophages. Sci Transl Med. 2022;14:eabo2952.
7. Colotti R, Bastiaansen JAM, Wilson A, Flogel U, Gonzales C, Schwitter J, Stuber M, van Heeswijk RB. Characterization of perfluorocarbon relaxation times and their influence on the optimization of fluorine-19 MRI at 3 tesla. Magn Reson Med. 2017;77:2263–2271.
8. van Heeswijk RB, Colotti R, Darçot E, Delacoste J, Pellegrin M, Piccini D, Hernando D. Chemical shift encoding (CSE) for sensitive fluorine-19 MRI of perfluorocarbons with complex spectra. Magn Reson Med. 2018;79:2724–2730.
9. Ludwig KD, Hernando D, Roberts NT, van Heeswijk RB, Fain SB. A chemical shift encoding (CSE) approach for spectral selection in fluorine-19 MRI. Magn Reson Med. 2018;79:2183–2189.
10. Darçot E, Yerly J, Hilbert T, Colotti R, Najdenovska E, Kober T, Stuber M, van Heeswijk RB. Compressed sensing with signal averaging for improved sensitivity and motion artifact reduction in fluorine-19 MRI. NMR Biomed. 2021;34:e4418.
11. Darçot E, Colotti R, Brennan D, Deuchar GA, Santosh C, Heeswijk RB. A characterization of ABL‐101 as a potential tracer for clinical fluorine‐19 MRI. NMR Biomed. 2020;33:e4212.